(3/3) Women in developing countries are disproportionately affected by cervical cancer due to a lack of access to preventative medicine – innovations in nano-based cancer therapies are paving the way for a cancer-free future. By Mia Shepherd.
The third article of our November '23 feature on Cancer (3/3)
Cervical cancer is one of the leading causes of cancer-related deaths in women worldwide. In 95% of cases, it is caused by the human papillomavirus (HPV), one of the most common sexually transmitted infections. It infects cells in the cervix, resulting in abnormal growth and ultimately leading to cancer. This global health issue significantly affects women in low and middle-income countries (LMICs) with limited access to screening, vaccines, and treatment. In 2020, 90% of cervical cancer deaths occurred within these regions, with a mortality rate of over 200,000 per year. Researchers around the world are exploring the benefits of nanotechnology to develop vaccines against cervical cancer – none of which have been approved yet, however promising candidates characteristic with good safety and tolerability profiles have been identified preclinically.
The HPV vaccine can prevent most cases of cervical cancer if it is given to girls and women before they are exposed to the virus. Vaccine programs in the UK have decreased incidence rates by 86% between 2010-2016. However, in cases where cancer does develop, the current standard of care is surgery, radiation, and chemotherapy. Not only are these solutions accompanied by serious side effects, but access is often limited in LMICs, and even then treatment is not always effective. Chemotherapy specifically, is infamous for its lack of selectivity when targeting cancerous cells, resulting in unwanted side effects, and possible drug resistance. Given the invasiveness and limitations of available conventional therapies, it is clearly necessary to develop novel treatments to reduce the incidence and mortality due to cervical cancer. One approach is to develop vaccines that can be given preventatively or as a treatment for both HPV and cervical cancer, enhanced by the technological capabilities of the nano toolbox.
Most available vaccines are made from inactive pathogens that stimulate the body’s immune system to respond, and develop long-lasting memory cells. However, this approach is less effective in cases where the immune response is too weak to provide protection. Another disadvantage is that such pathogens can mutate in the body, and revert to their pathogenic form. Artificial nanomaterials can deliver antigens (biomolecules that our immune systems recognise as foreign, but cannot cause disease itself) to specific cells, which can trigger powerful immune responses, killing cancer cells. Nanotechnology can be used to overcome the limitations of traditional vaccines: the risk of reversion to pathogenic form and mutation, to enhance the effectiveness of immunotherapies.
Liposome-based nanovaccines in cervical cancer
One approach uses liposomes (nanoparticular capsules that can interface with cell membranes) to encapsulate and deliver drugs and antigens. The structure of a liposome and antigen attachment/ encapsulation is shown in Figure 1. Liposomes protect antigens from degradation in the body, enabling their controlled release at the target. Not only does controlled release avoid the damage of healthy tissue, it also enhances the body’s immune response, reducing and possibly eliminating the need for booster immunisations.
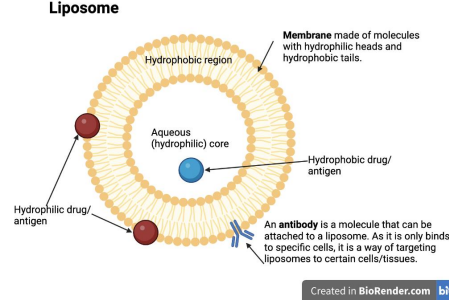
More recently, research into combining liposomes with other technologies such as gene therapy has begun. A research group in Iran led by Dr Hesam Karimi has developed a nanovaccine that uses liposomes to expose mutated HPV genes to eradicate the viral proteins found inside cells. Their results proved that combination therapy promoted a strong and long-lasting immune response, which even at low doses had both preventative and therapeutic effects on tumours in mice. Dr Karimi stated “it is really fascinating to see how these various nanoagents would interact with the immune system” seeing as it is “a complex but intriguing system”.
Another study published in late 2022 by researchers at the Xi’an Jiaotong University in China used liposomes to deliver the CRISPR/Cas9 gene editing system to cancer cells. They elicited highly effective antitumour effects by targeting viral genes encoded with HPV proteins. CRISPR/Cas9 is an enormously powerful tool, although delivering it to target sites in the body poses a major challenge in realising its therapeutic potential. Certain barriers like the cell membrane can prevent entry to the cell, allowing the immune system to recognise it as foreign, and initiating an inflammatory response. The results of this study may represent a significant step towards enabling the translation of CRISPR/Cas9.
Another group of scientists at Zhejiang University in China engineered a nanovaccine by combining nanotechnology and photodynamic therapy to stimulate the antitumour activity of immune cells. Made from encapsulated antigens and photosensitisers, the vaccine responds to infrared laser irradiation, enabling the controlled and targeted release of antigens. Positive results seen in mice, lead researchers to “hope that [their] research can provide a theoretical basis for the development of therapeutic vaccines that can be applied in the clinic”.
Obstacles and future directions
Few barriers between translating nanovaccines to the clinic remain, including standardisation in research, safety, and costs. The optimal size of antigen-delivering nanocarriers largely depends on the chemical properties of the materials being used and their specific applications. By developing a standardised method and criteria, scientists can choose appropriate nanomaterials for different contexts, which is likely to be more beneficial, compared to a one-size-fits-all silver bullet.
The application of nanomedicine is hindered by the lack of standardised research guidelines and protocols. Professor Morteza Mahmoudi and his group in Michigan State University published findings that, “reveal an extreme lack of consistency in the analyses researchers use to understand how nanomedicines work in the body”. This leaves room for doubt about the “effectiveness and safety of treatments based on nanomedicines”. Mahmoudi is calling for the standardisation of the field by “modifying study protocols, methodologies, and analytical techniques” to improve the safety and efficacy of nanomedicines.
Regarding safety and cost, it is worth investigating whether it is possible to develop technology that tracks the long-term effects of nanovaccines in the body. The novelty of nanovaccines relative to conventional vaccines, means stringent safety testing is required to establish risk profiles. Considering cervical cancer has a higher mortality rate in LMICs, novel nanovaccines must be relatively cheap, easy to store and transport, and easily scaled-up. Otherwise, the impact of this technology might fail to make a significant impact in places where they are needed the most.
Despite the challenges, the prospects of using nanovaccines as a strategy to deal with HPV and cervical cancer are hopeful. Recent studies have shown promising results as researchers continue to develop safe, effective, and globally accessible nanovaccines to save lives around the world. The progress being made underscores the importance of continued investment and collaboration in the battle against cervical cancer.
References:
Cover Image: V. Rajavat, UCL Science Magazine Graphic Designer
In 95% of cases: Cervical cancer, World Health Organisation, [Accessed 22/11/2023]
200,000 per year: Theivendren, P., Hegde, Y. M., Srinivas, G., Palanivel, M., Shanmugam, N., Kunjiappan, S., Vellaichamy, S., Gopal, M., & Dharmalingam, S. R. (2023). ‘A recent advancement in nanotechnology approaches for the treatment of cervical cancer’, Anti-Cancer Agents in Medicinal Chemistry, 23(1), 37–59. doi.org/10.2174/1871520622666220513160706
Decreased incidence rates by 86%: Mesher, D., Panwar, K., Thomas, S. L., Edmundson, C., Choi, Y. H., Beddows, S., & Soldan, K. (2018). ‘The impact of the national HPV vaccination program in England using the bivalent HPV vaccine: Surveillance of type-specific HPV in young females, 2010–2016’, The Journal of Infectious Diseases, 218(6), 911–921. doi.org/10.1093/infdis/jiy249
Revert to their pathogenic form: Lee, S. W., Markham, P. F., Coppo, M. J., Legione, A. R., Markham, J. F., Noormohammadi, A. H., Browning, G. F., Ficorilli, N., Hartley, C. A., & Devlin, J. M. (2012). ‘Attenuated vaccines can recombine to form virulent field viruses’, Science (New York, N.Y.), 337(6091), 188. doi.org/10.1126/science.1217134
Preventative and therapeutic effects on tumours: Karimi, H., Soleimanjahi, H., Abdoli, A., & Banijamali, R. S. (2020). ‘Combination therapy using human papillomavirus L1/E6/E7 genes and archaeosome: A nanovaccine confer immune adjuvanting effects to fight cervical cancer’, Scientific Reports, 10(1). doi.org/10.1038/s41598-020-62448-3
Targeting viral genes encoded with HPV proteins: Zhen, S., Qiang, R., Lu, J., Tuo, X., Yang, X., & Li, X. (2022). ‘CRISPR/cas9‐hpv‐liposome enhances antitumor immunity and treatment of HPV infection‐associated cervical cancer’, Journal of Medical Virology, 95(1). doi.org/10.1002/jmv.28144
Controlled and targeted release of antigens: Zhang, L., Wang, K., Huang, Y., Zhang, H., Zhou, L., Li, A., & Sun, Y. (2022). ‘Photosensitizer-induced HPV16 E7 nanovaccines for cervical cancer immunotherapy’, Biomaterials, 282, 121411. doi.org/10.1016/j.biomaterials.2022.121411
Optimal size of antigen-delivering nanocarriers: Azharuddin, M., Zhu, G. H., Sengupta, A., Hinkula, J., Slater, N. K. H., & Patra, H. K. (2022). ‘Nano Toolbox in immune modulation and nanovaccines’, Trends in Biotechnology, 40(10), 1195–1212. doi.org/10.1016/j.tibtech.2022.03.011
“Effectiveness and safety of treatments based on nanomedicines”: Ashkarran, A. A., Gharibi, H., Voke, E., Landry, M. P., Saei, A. A., & Mahmoudi, M. (2022). ‘Measurements of heterogeneity in proteomics analysis of the nanoparticle protein Corona Across Core Facilities’, Nature Communications, 13(1). doi.org/10.1038/s41467-022-34438- 8