Since the 20th century, the issue of antimicrobial resistance has developed into a gordian knot of gargantuan proportions. This has inspired research into treatments from other medical fields in hopes of minimising the consequences of antibiotic resistance. By James Lau Yi Wei
Our modern antibiotic era can be traced back to the idea of a “magic bullet”, first conceived by German physician Paul Ehrlich in 1907. This therapeutic concept postulates a "bullet” that selectively exterminates harmful bacteria while sparing human cells. Alexander Fleming’s subsequent discovery of the most famous antibiotic penicillin in 1928 was a pivotal milestone that gained traction, leading to the antibiotic revolution. This was a massive boom in antibiotic discovery between the 1950s and 1970s. Heavy inspiration was drawn from nature, leading to the creation of many antibiotic classes that are now widely known and studied. Unfortunately since, antibiotic drug discovery has tapered off; fewer of such drug classes have been developed. [1] In recent times, overwhelming evidence of a reduction in antibiotic efficacy has led the World Health Organisation to issue a warning of an impending “post-antibiotic era”. [2]
Antibiotic resistance arises from the overprescription, perhaps even the abuse, of antibiotics that treat infections in livestock and humans. Consequently, bacteria have developed a wide array of simple counter mechanisms at both the cellular level and community level to oppose antibiotic action. On the cellular level, bacteria cells may synthesise different biological molecules to inhibit the pharmacological activity of antibiotics. One notable adaptation is the β-lactamase enzyme that hydrolyses the β-lactam ring functional group found in several antibiotic drug classes. This heterocyclic ring is the pharmacophore of these drug classes – the essential component that disrupts bacterial cell wall synthesis. Its inactivation curtails the therapeutic effect of the drug. On the community level, bacterial colonies may form biofilms on many different surfaces composed of various biological components. This barrier surrounds the colony to physically restrict the entry of antibiotics and promote the transfer of antibiotic resistant genes between individual bacteria. [3]
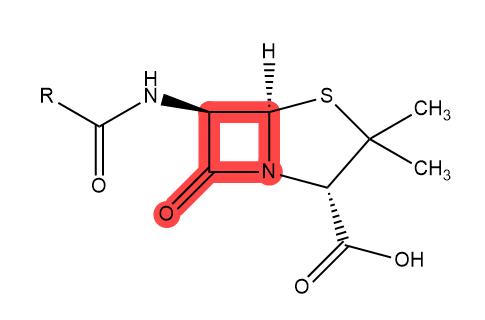
Despite persistent efforts in research and development, bacteria are still likely to develop resistance to newly discovered classes of antibiotics. In 1996, Linezolid was the first antibiotic of the oxazolidinone class to be discovered. Entering the market in 2000, it is licensed to treat infections caused by antibiotic-resistant strains of bacteria including Methicillin-Resistant Staphylococcus aureus (MRSA). [4] Linezolid binds to the 50S ribosomal subunit found only in bacteria, responsible for the translation of mRNA to protein for cell growth. The pharmacophore that enabled binding was identified to consist of a (s)-2-oxazolidinone ring, an acyl aminomethyl group and an aryl group. [5] At the onset of its introduction, it was assumed that linezolid’s unique mechanism of action meant that “antibiotic resistance would be rare and difficult for bacteria to develop”. [4] However, rRNA mutations within ribosomal subunits may alter the shape of the binding site to effectively resist linezolid and other oxazolidinones, resulting in cases of resistance to Linezolid becoming more common, especially in Mycobacteria species. [6] Although the specific pharmacological action of antibiotics is important in preventing collateral damage to human cells, this could be rendered obsolete via bacterial adaptation mechanisms. It is unfortunate that the current strategy of antibiotic dependence appears to be a Sisyphean endeavour.
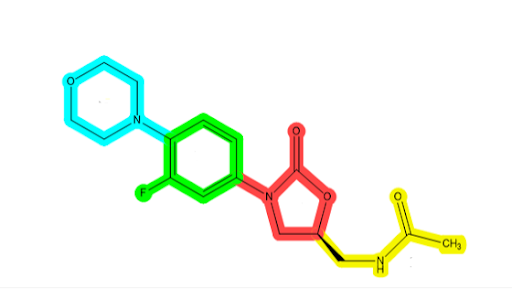
Other fields of medicine could provide a potential solution to this predicament. Photodynamic Therapy (PDT) is a popular treatment option for certain cancer types, which could be repurposed for antibacterial treatment. PDT has three components: a photosensitiser drug, light and molecular oxygen. After the photosensitiser is injected into the patient, exposure to a specific wavelength of light, induces electron donation from the photosensitiser to oxygen molecules. The generation of reactive oxygen species (ROS) causes widespread oxidative damage, resulting in tumour cell death. [7] In a research paper [8], scientists created a photosensitiser drug which possesses a mechanism that selectively targets bacteria. This was achieved through in-situ polymerisation of polymethylene blue (photosensitiser) onto a bacterial cellulose fibre network (the carrier) to form a composite material known as Bacterial Cellulose-Polymethylene Blue (BC-PMB). BC-PMB allows targeting of bacteria on both community and cellular levels, complicating the development of resistance mechanisms.
As the safety, efficacy, formulatability, and pharmacokinetics of BC-PMB for intravenous use have not yet been determined, more realistic applications of BC-PMB could be explored to halt the spread of antibiotic-resistant bacteria. A BC-PMB coating could be applied onto hospital furniture and medical equipment to hinder the spread of healthcare-acquired infections. BC-PMB could also be added to water and air filtration systems such that these can capture bacteria. Regardless, these are subjected to the relevant laboratory tests and regulatory requirements.
Although the “post-antibiotic era” has not arrived, antibiotic resistance growth could soon outpace antibiotic development. Development of these classes occur much slower than other drugs, taking over 20 years, compared to the typical 15. Moreover, the high costs ($568-700 million) associated with drug development further disincentivise pharmaceutical firms. [9] As such, a paradigm shift may be necessary to advocate for the development of unconventional antibacterial treatments like BC-PMB.
References:
Cover Image: V. Rajavat, UCL Science Magazine Graphic Designer
[1] Aminov RI. A brief history of the antibiotic era: lessons learned and challenges for the future. Front Microbiol. 2010;1:134. [Accessed on: 10/10/2023]. Available from: https://pubmed.ncbi.nlm.nih.gov/21687759/.
[2] World Health Organisation. Antibiotic Resistance [Internet]. World Health Organisation. 2020. [Accessed on: 26/10/2023]. Available from: https://www.who.int/news-room/fact-sheets/detail/antibiotic-resistance.
[3] Domenico Schillaci, Virginia Spanò, Barbara Parrino, Anna Carbone, Alessandra Montalbano, Paola Barraja, Patrizia Diana, Girolamo Cirrincione, and Stella Cascioferro “Pharmaceutical Approaches to Target Antibiotic Resistance Mechanisms”. Journal of Medicinal Chemistry 2017 60 (20), 8268-8297. [Accessed on: 12/9/2023].Available from: https://pubs.acs.org/doi/10.1021/acs.jmedchem.7b00215.
[4] Long Katherine S., Vester Birte. “Resistance to linezolid caused by modifications at its binding site on the ribosome.” Antimicrob Agents Chemother. 2012 Feb;56(2):603-12. [Accessed on: 13/10/2023]. Available from: https://www.ncbi.nlm.nih.gov/pmc/articles/PMC3264260/.
[5] 1.Foti C, Piperno A, Scala A, Giuffrè O. Oxazolidinone Antibiotics: Chemical, Biological and Analytical Aspects. Molecules [Internet]. 2021 Jul 14 [cited 2022 May 11];26(14):4280. Available from: https://www.ncbi.nlm.nih.gov/pmc/articles/PMC8305375/
[6] Gan WC, Ng HF, Ngeow YF. Mechanisms of Linezolid Resistance in Mycobacteria. Pharmaceuticals (Basel). 2023 May 24;16(6):784 [Accessed on: 13/10/2023]. Available from: https://www.ncbi.nlm.nih.gov/pmc/articles/PMC10303974/
[7] Correia JH, Rodrigues JA, Pimenta S, Dong T, Yang Z. Photodynamic Therapy Review: Principles, Photosensitizers, Applications, and Future Directions. Pharmaceutics. 2021 Aug 25;13(9):1332.[Accessed on: 7/11/2023]. Available from: https://www.ncbi.nlm.nih.gov/pmc/articles/PMC8470722/.
[8] Zhao J, Guo XY, Yang J, Xie Y, Zheng Y. In Situ Polymerization of Methylene Blue on Bacterial Cellulose for Photodynamic/Photoelectricity Synergistic Inhibition of Bacterial Biofilm Formation. ACS Applied Materials & Interfaces. 2023 Sep 8;15(37):43591–606. [Accessed on: 14/9/2023]. Available from: https://pubs.acs.org/doi/10.1021/acsami.3c09449
[9] PhRMA. Nearly 90 Medicines in Development for Against Drug-Resistant Infections [Internet]. phrma.org. [Accessed on: 29/10/2023]. Available from: https://phrma.org/en/resource-center/Topics/Medicines-in-Development/Medicines-in-Development-for-Antimicrobial-Resistance-2021-Report.